With the dominance of lithium-ion batteries in the market today, the question arises: will we ever run out of lithium?
The battery value chain is estimated to grow by over 30 percent annually from now until 2030. And the demand for battery factories is expected to grow from 700 GWh in 2022 to 1.7 TWh by 2025, not only in China where the chain segments are mature, but also in the EU and the United States with efforts towards localising supply chains. But forecasts have indicated a near-term supply deficit in lithium as early as 2025, in part due to the rising sale of EVs.
To anticipate this evolution and support the growing need for batteries across industries, from EVs to consumer electronics to defence, manufacturers are diversifying their cell chemistry.
This search for new chemistries is driven by the demand for increased energy density, cost competitiveness, safety and lifespan. Besides materials improvement, the battery management system is also adopting new technologies to better monitor and regulate the battery in real-time.
Here, we explore and compare some of the most promising emerging battery chemistries, plus, the secret to extracting maximum value from them.
An evaluation of emerging chemistries
Every new chemistry comes with its specific strengths and weaknesses. While the most common battery chemistry today is graphite for anodes and lithium nickel manganese cobalt oxide (NMC) or lithium iron phosphate (LFP) for cathodes, new chemistries are well under development.
The figure below shows the progress and evolution of chemistry development over the past 20+ years.
The Volta Foundation's 2023 Battery Report. The diagram shows the timeline for new cell chemistry development from 2000-2023. (Source: The Volta Foundation)
There are four emerging chemistries that show the most promise today:
- Silicon anode: After years of incremental improvement, silicon is now a mainstay anode material in batteries in both consumer electronics and automotive sectors. It has a theoretical capacity 10 times larger than that of graphite but has poor lifespan due to the large volume expansion of over 300% during lithiation. Regardless of the cycle life issues, silicon has been gaining popularity since 2022, due to the 20–40% energy density boost it provides, in addition to its abundancy and lower carbon footprint. The market size is expected to grow from $2 billion by 100 times from now to 2035.
- High-nickel cathodes: For cathodes, high-nickel layer oxide is sought after for enhancing energy density towards 800 Wh/kg, coupled with the incentive to reduce cost and the controversial mining of cobalt. The common compositions are NCM-811 (LiNi0.8Co0.1Mn0.1O2) and NMC (LiNi0.8 Co0.15Al0.05O2). The disadvantage is the trade-off in rate capability because of lowered cathode structural stability with reduced cobalt content. Nowadays both high-nickel compositions are largely deployed in the automotive industry.
- Solid-state batteries: The solid-state battery was first commercialised in 2015, and since then faced both excitement and scepticism in its development. This is sometimes referred to as a lithium metal battery because replacing the liquid electrolyte with a ceramic or solid polymer allows the lithium metal to accumulate directly on the anode.
The huge energy-density increase solid-state batteries deliver is the main driver to launch this chemistry, for higher range and longer runtime. The reduced risk of a battery fire due to the absence flammable liquid electrolyte in the event of mechanical damage is another bonus. The technology challenge remains at the interfaces between the anode and the solid electrolyte, where dendrite formation, poor contact and cracking can lead to battery failure. Toyota in 2023 announced mass production of solid-state batteries by 2027/28 with sulphide-based electrolytes while some other major players continue to be wary of the long development time and high production cost.
- Sodium-ion batteries: Sodium, as an alternative to lithium, has the advantage of being naturally abundant, with a sustainable supply available from seawater. In 2023, CATL announced the first sodium-ion battery to power Chery EV, with Prussian white cathode and hard carbon anode. Despite the issue of low energy density, many large manufacturers like BYD and Northvolt have followed the pursuit of full-scale production of sodium-ion batteries because of the 30% reduction in cost compared to lithium-ion batteries.
- Hybrid pack design: With the availability of multiple battery chemistries in the market also comes hybrid pack design. The hybrid approach mixes cells of different chemistries in a pack. For example, CATL and BYD used a hybrid of sodium-ion and lithium-ion batteries when adopting sodium-ion technology in mass-production vehicles to mitigate the range deficit. NIO also launched its 75 kWh hybrid-cell battery with both NMC and LFP to improve cold temperature range. However, the hybrid trend demands additional technology to provide accurate state estimation and precise control algorithms for ensuring both performance and safety.
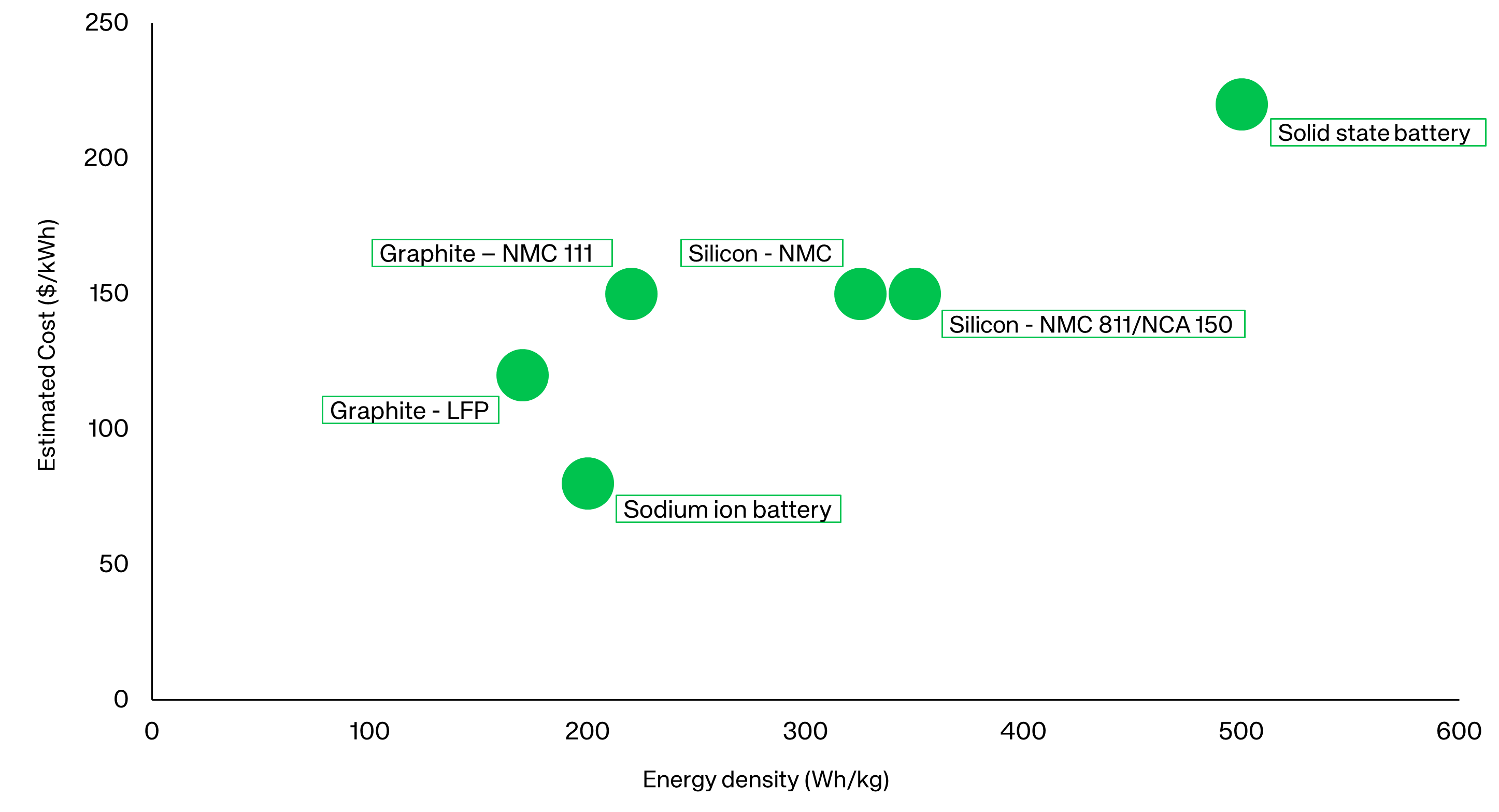
Comparison of cost and energy density for different chemistry options (Source: Breathe Battery Technologies)
Top tip: For a comprehensive comparison of battery chemistries against a full list of performance metrics, check out the Volta Foundation’s 2023 Battery Report, page 115.
Extracting value from emerging chemistries
As with lithium-ion, there are always additional ways to extract value from the battery. While it’s all well and good to explore different chemistries as a means of achieving greater performance, each chemistry - and even different batteries using the same chemistry - has a wide range of potential energy density, power density, and cycle-life available. This leaves a significant opportunity on the table to optimise battery performance. Advanced battery control technology is increasingly being implemented by EV and consumer electronics OEMs as a means of extracting maximum performance from available chemistries. This technology ensures optimal charge and discharge cycles, in different usage patterns, to extract more energy and lifetime from each battery.
Regardless of the material, it is important to understand the mechanisms happening inside the battery. Advanced control algorithms, specifically those that use a real-time physics-based model running online, can easily adapt to new battery chemistries due to the underlying physical models being formulated based on the kinetics and thermodynamics of electrochemical reactions.
Most batteries, comprised of an anode, a cathode and the electrolyte, share large similarities in the kinetics and thermodynamics underpinning their performance and longevity. As a result, control strategies informed by physics offer the versatility to be applied to multiple chemistries to extract maximum potential.
Adopting new chemistries without the addition of advanced battery control technology will always leave the battery underutilised because today’s standard control strategies are too rudimentary to exploit the strengths and weaknesses of these chemistries based on their underlying physics. Whether the future of battery chemistry stays with lithium-ion, or advances to one of the alternatives mentioned above, we should not rely on chemistry alone to achieve a step-change in battery performance. OEMs should always explore how complimentary technology can help them achieve their battery and user experience objectives.
So, are emerging chemistries a suitable alternative to today’s lithium-ion batteries?
The answer is still uncertain. But with a synergistic approach between materials improvement and software development, we can make significant advancements to the performance of emerging battery chemistries despite their different electrochemical reactions and degradation mechanisms. What is clear, is that a one-size-fits-all approach to battery control is no longer good enough to extract all available performance, regardless of chemistry.
The combination of materials and software-based control strategies is going to be critical in achieving improved battery efficiency, safety and lifetime, and collaboration between battery makers, software suppliers and OEMs is essential to create a more sustainable future powered by more performant battery solutions.